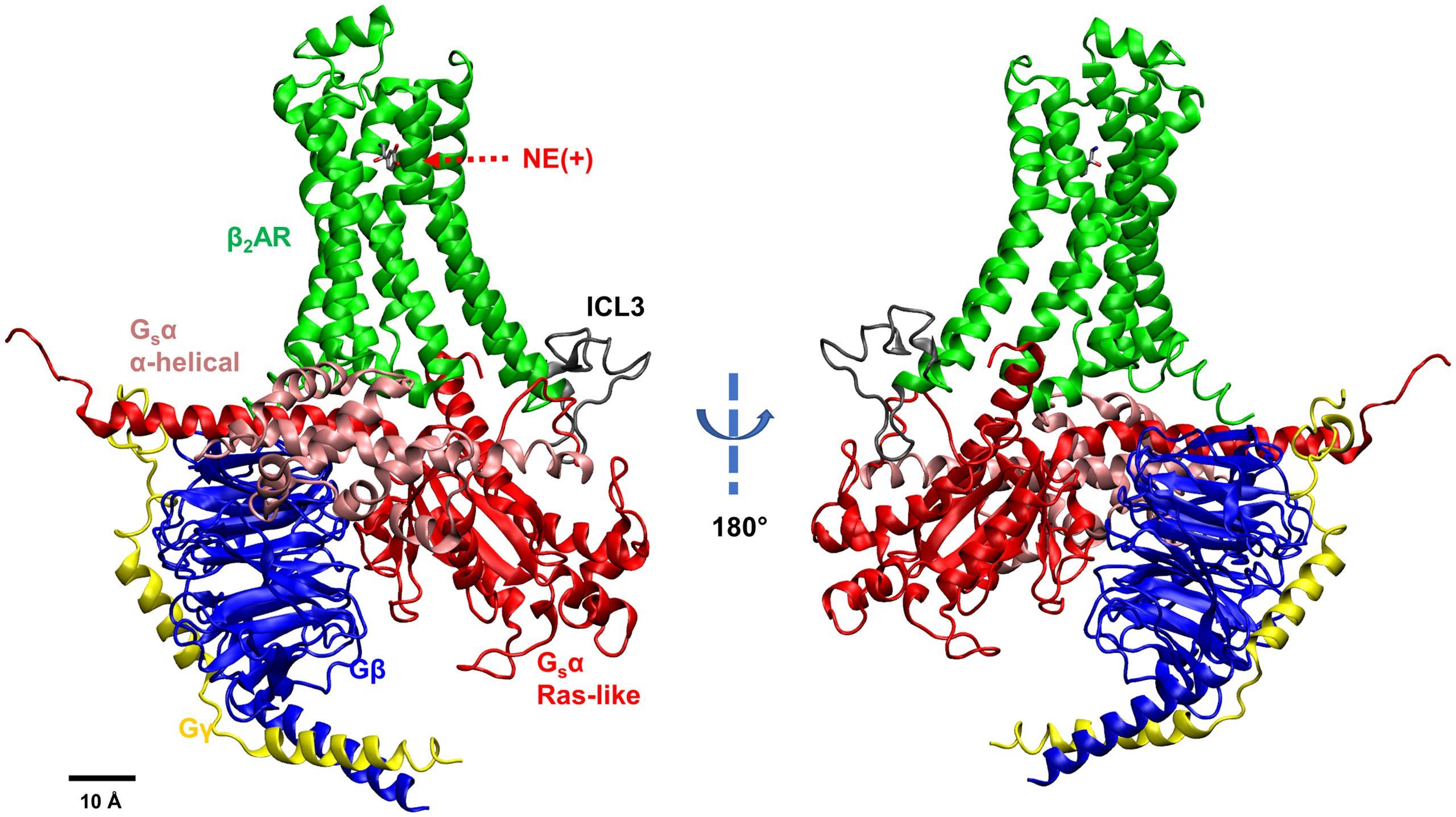
Snapshot of the β2AR–Gs system: NE(+)-bound β2AR coupled with Gs protein. Different subunits and loops are illustrated by different colors (Green – β2AR, Gray – intracellular loop 3 or ICL3, Pink – GsαAH domain, Red – GsαRas domain, Blue – Gβ, Yellow – Gγ). From Y. Han, et al., “Elucidation of a dynamic interplay between a beta-2 adrenergic receptor, its agonist, and stimulatory G protein,” The Proceedings of the National Academies of Sciences, vol 120, no. 10, 2023.
Interactions not before seen between medically important beta-adrenergic receptor and its associated G protein offer clues to better heart, lung, and other medications.
Cell-surface receptor proteins and their associated G proteins lie at the center of many medically important processes and drugs. To better understand how they work, a team from the University of California, Davis (UC Davis), used the Anton 2 supercomputer designed and developed by D. E. Shaw Research (DESRES), and hosted at PSC, to simulate a G protein and its interactions with a cell-surface beta-adrenergic receptor protein known to trigger its activity. These simulations revealed movements in both molecules that had not been seen before. The results offer clues to designing drugs to better target G protein activity to treat specific diseases.
WHY IT’S IMPORTANT
The G proteins are linchpins in our cells’ response to signals from elsewhere in the body. Molecular messengers convey these signals by first sticking to cell-surface proteins called G protein-coupled receptors (GPCRs). By changing its shape, the GPCR then passes the message along to an attached G protein, inside the cell. The activated G protein then passes it to hundreds to thousands of systems inside the cell, altering its behavior.
GPCRs and G proteins are medically crucial — they’re the targets for one third to one half of all drug therapies, directly or indirectly. An improved understanding of how they interact could open new and better ways for medications to treat conditions as different as heart disease, impaired breathing conditions, migraine, and even anxiety disorders.
“There are now multiple [X-ray crystallography] structures of GPCR and G protein complexes available. The problem is these proteins are highly dynamic [in a way these structures don’t capture]. Using molecular dynamics simulations, we can study how … the ligand binding can [change] their conformations.” — Igor Vorobyov, UC Davis
GPCRs and G proteins, though, are difficult to study in the lab. Like restless toddlers, they will not sit still. Methods like X-ray crystallography, which take snapshots of molecular structures, don’t work particularly well on them because some of the most interesting parts of the proteins move too much to be imaged.
Yanxiao Han, a postdoctoral scholar working in the lab of Igor Vorobyov at UC Davis, wanted to find out more about how GPCRs and G proteins move while they’re connected and communicating with each other. Working with colleagues there and at American River College, Sacramento, and the VA Northern California Health Care System, she began her work with powerful traditional supercomputers at the Texas Advanced Computing Center and the San Diego Supercomputer Center, PSC’s partners in the NSF-funded ACCESS program. To take the work to the next level, though, she would need to carry out simulations at far longer timescales than possible with traditional supercomputers. She thus turned to Anton 2, a special-purpose supercomputer for molecular dynamics (MD) simulation, which is made available without cost by DESRES and hosted at PSC with operational funding from the National Institutes of Health.
HOW PSC HELPED
Previous lab and simulation work, including those by Han and others on traditional computers, had painted a picture of how GPCRs and G proteins affect each other in a broad sense. First, a messenger molecule specific to a given GPCR sticks to the receptor on the outside surface of the cell membrane. The receptor, a large protein that spans the membrane, then changes its shape. On the inside of the cell, that change alters how it connects with a G protein. The G protein shifts its shape in turn, separating from the GPCR and moving along the membrane’s inner surface to relay the signal to many of the cell’s systems. This alters how the cell behaves.
Scientists knew that the G protein could exist in two states — “closed,” which carries a signal, and “open,” which does not. In all the static structures researchers had been able to produce, the receptor-bound G protein was open. No one knew how or when the protein closed and activated. Also worrisome to scientists was the fact that part of the GPCR near the G protein, called the intracellular loop 3, moved so much that no structures had ever been able to capture it — it was basically invisible.
In Han’s earlier simulations, the protein hadn’t fully “settled in.” With the limited simulated times available via general-purpose supercomputers, she simply wasn’t getting the whole story. DESRES designed and constructed the Anton supercomputer to vastly accelerate the process of MD simulations. Because of its special-purpose architecture, Anton 2 can simulate the behavior of the GPCR and G protein on timescales as much as 50 times longer than general-purpose supercomputers can in a given amount of time.
“With Anton 2, we can get 5-microsecond trajectories in a few days. Using [traditional supercomputers], we can only get 20- to 30-nanosecond trajectories in a day and cannot clearly see the conformational transition.” — Yanxiao Han, UC Davis
Han simulated a GPCR called the beta-2 adrenergic receptor (β2AR). They looked at its movements while bound to its natural ligand, a messenger molecule called norepinephrine, as well as the stimulatory G protein (Gs). This system is part of the body’s “flight or fight” response, in which norepinephrine signals the heart to beat faster and harder, among many other responses. β2AR is the target of many important drugs, including those for treating asthma, emphysema, and congestive heart failure.
In Han’s simulations, norepinephrine-bound β2AR caused the Gs protein to close before detaching from the receptor. That hadn’t been seen either in previous static structures or simulations. More interesting, she found that intracellular loop 3’s movement was key to the Gs protein activating — a result that hadn’t been predictable before because of its invisibility in earlier structures. She, Vorobyov, and their collaborators reported their results in the prestigious Proceedings of the National Academy of Sciences USA in February 2023
The work is part of a larger effort by an extended team of scientists to understand how many cells and tissues communicate at once, to shed light on large problems like controlling heart failure or arrhythmias. The team has begun simulating another important type of GPCR, the beta-1 adrenergic receptor, to see how its communication with G proteins may differ from β2AR — and so how drugs can be tailored to affect one GPCR or another more specifically.