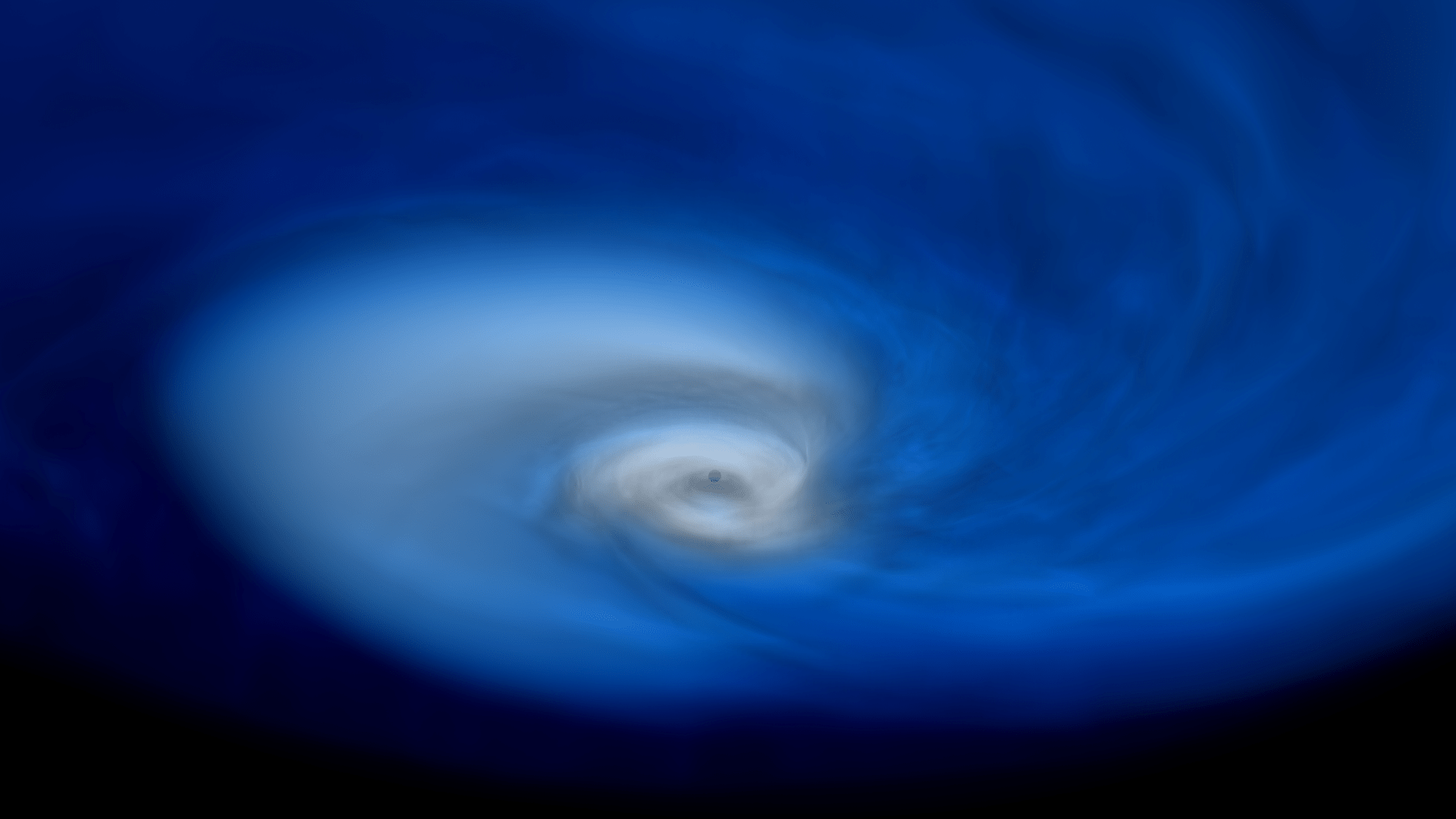
The two, massive neutron stars merge with a great gravitational clang — but little electromagnetic signal gets out.
Scientists had not predicted absence of electromagnetic signal in 2019 detection
A collision of two massive neutron stars detected by gravity-wave observatories in 2019 produced no observed flash of ultraviolet light. This flew in the face of both an earlier neutron-star-merger detection and computer simulations. An international team led from the Pennsylvania State University used PSC’s Bridges-2 system, among others, to carry out simulations of larger neutron-star mergers that suggest such collisions will produce a much smaller electromagnetic signal than previously expected. This could explain the lack of a UV detection in 2019 and offers predictions for new telescopes to test.
WHY IT’S IMPORTANT
There aren’t many natural phenomena as dramatic as two massively dense, collapsed stars smashing into each other. It’s a puzzling fact, though, that some of these events proceed with a whimper rather than a bang. Gravity-wave-sensitive instruments can now detect when black holes or neutron stars collide. But sometimes, these collisions result in no electromagnetic “flash” that our most sensitive telescopes can detect.
The GW190425 event, detected in 2019, was the second merger of two neutron stars revealed by the Advanced LIGO and Advanced Virgo gravity wave detectors. Unlike the 2017 GW170817 neutron-star-merger event, though, GW190425 wasn’t accompanied by an ultraviolet-light flash that astronomers could find. Scientists wondered whether they couldn’t find a UV signal because the telescopes weren’t quite aiming at the right place to see it, or because there wasn’t one.
“We wanted to model the particular event, GW190425, detected by the LIGO and Virgo observatories … One of the things we asked ourselves … was why was there no [electromagnetic] counterpart? Was it because there was nothing to find? Or was it because we didn’t search deeply enough, or because we didn’t cover the right area in the sky?” — David Radice, Pennsylvania State University
David Radice of the Pennsylvania State University and colleagues there and at institutions in Germany and Italy wanted to figure out which of these scenarios was true. They started with computer simulations of neutron-star mergers using a number of supercomputers at facilities in the U.S. and Europe. A key computing resource in their work was PSC’s Bridges-2 advanced research computer.
HOW PSC HELPED
The first clue was that “dark” GW190425 was actually larger than flashy GW170817.
Neutron stars are the product of giant stars exploding. When one of these massive suns detonates, it blows much of its mass into space. But a collapsed star with roughly similar mass to that of our Sun remains. When the remnant star’s mass is larger than about 2.2 times that of the Sun, it rips a hole into spacetime and becomes a black hole. Less, and it becomes a neutron star. That’s an ultra-dense star composed of material that weighs about a million tons per teaspoon.
Gravitational wave detectors can tell astronomers the combined mass of these stars when they collide. In the case of the earlier, flashy GW170817 collision, that combined mass was 2.7 times that of the Sun. With the dark GW190425 collision, it was 3.4 solar masses, with one of the two participants possibly closer to the maximum size of a neutron star.
Judging from earlier simulations, GW190425 should have produced a visible UV flash. But the higher mass was an issue, because the earlier work had only simulated smaller mergers. Both “small” and “large” neutron-star collisions should result in a black hole, because in either case the new combined star would be greater than 2.2 solar masses. The larger collision, though, could possibly create a black hole large enough to swallow up much of the electromagnetic signal.
To test this idea, the team repeated earlier simulations, this time making the participating neutron stars larger. The massive simulations needed to be repeated many times. This was partly because the scientists only knew the combined mass of the GW190425 neutron stars. They needed to simulate different relative sizes for the two participants, ranging from equal size to one being 1.67 times as massive as the other. The latter is the maximum size the larger star could be without being a black hole, which could be ruled out by the gravity-wave-detection data.
“Bridges-2 is a very nice machine for us, because our code runs very well on this hardware … For neutron-star mergers in particular, we have to follow thousands of time scales. For this purpose, having fast single-core performance and having a good number of cores is essential.”
— David Radice, Pennsylvania State University
Bridges-2 was one of several systems the team used, including Comet at the San Diego Supercomputer Center and Stampede2 at the Texas Advanced Computing Center, PSC’s partners in the ACCESS network of NSF-funded supercomputers. Bridges-2 proved particularly good at these computations. Because of Bridges-2’s architecture, data moves very fast in and out of the computational cores. That greatly speeds the work when a simulation has to run for long times and be repeated many times, as the neutron-star merger sims did.
The simulations produced the surprising result that a larger neutron-star merger can be “quieter,” at least in terms of electromagnetic signal. Throughout the mass ratios of 1 to 1.67, the large mergers produced a smaller UV signal that wouldn’t have been detectable by today’s generation of telescopes. The astronomers hadn’t been aiming their telescopes in the wrong place to see GW190425; there just wasn’t anything for them to see.
The scientists are now waiting to see what future neutron-star mergers reveal. The Vera C. Rubin Observatory, currently being built in Chile and slated to begin survey work in 2024, will in theory be able to detect the faint UV flash predicted for larger neutron-star mergers. Such a detection would be a vital test of the computer simulations’ predictions. It would also provide real-world data that the scientists can fold back into their simulations to make them even more accurate.